Sergio de Lopez Diz, Roberto Martin Lopez, Francisco Javier Rodriguez Sanchez, Member IEEE, and Emilio Jose Bueno Peña, Senior Member IEEE.
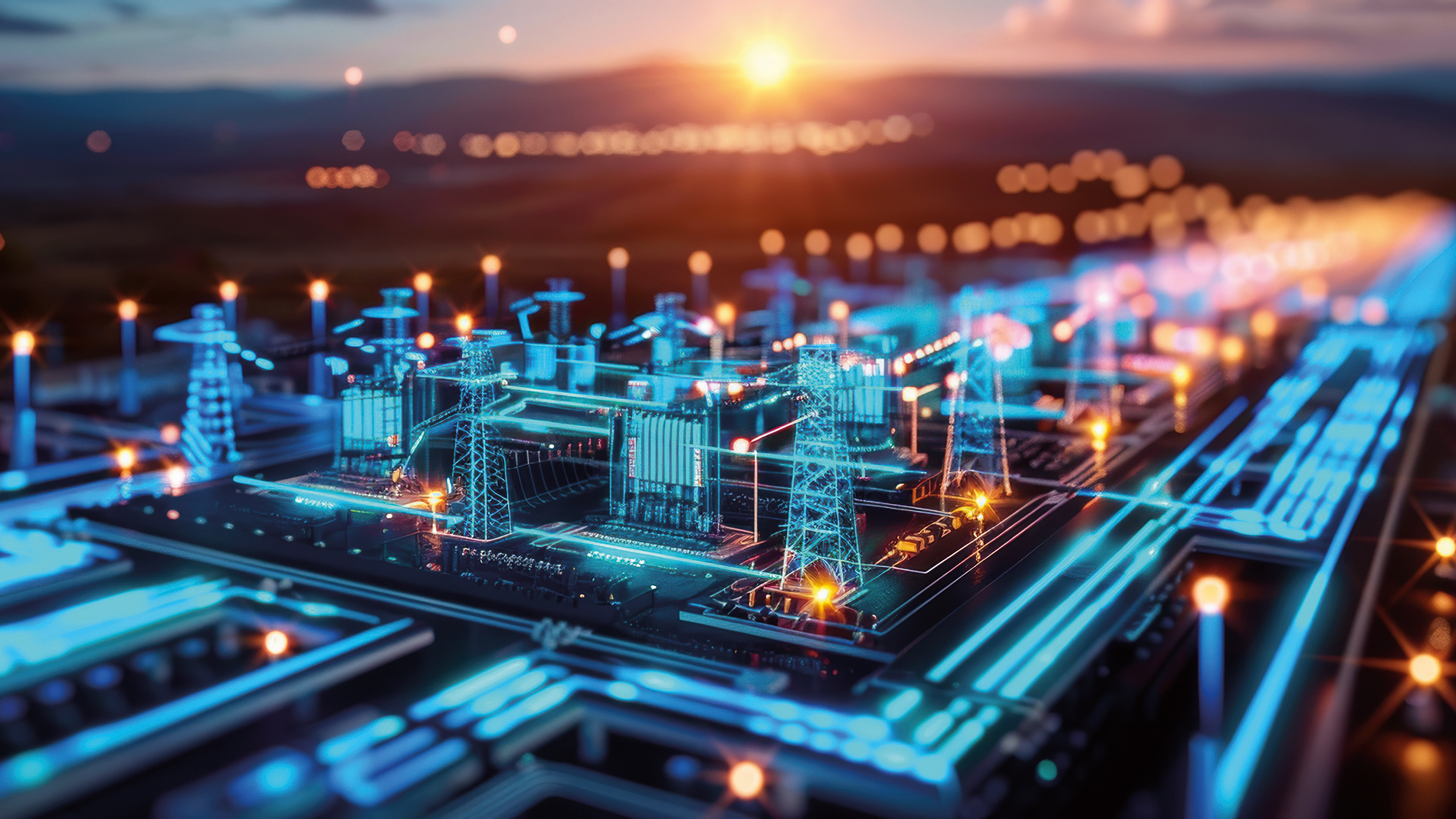
This IEEE Xplore paper from researchers at the Universidad de Alcalá, Spain, presents a comprehensive study on the application of Digital Twins (DT) for real-time, online impedance-based stability analysis in power distribution systems. The research explores how digital twins can be used to address stability challenges in power distribution, where advanced networks with multiple parallel inverters create complex dynamics that require precise monitoring and control.
Challenges for the stability of power distribution systems
In modern power systems, particularly those integrating renewable energy sources, many inverters often operate in parallel within a network, generating stability concerns due to dynamic interactions. This paper’s main focus is on improving the real-time stability of three-phase AC systems through small-signal analysis, which involves examining the impedance interactions between power sources and loads. Typically, the stability of these systems is assessed by calculating the source-to-load impedance ratio in the dq plane, using the Generalized Nyquist Criterion (GNC) as a stability metric.
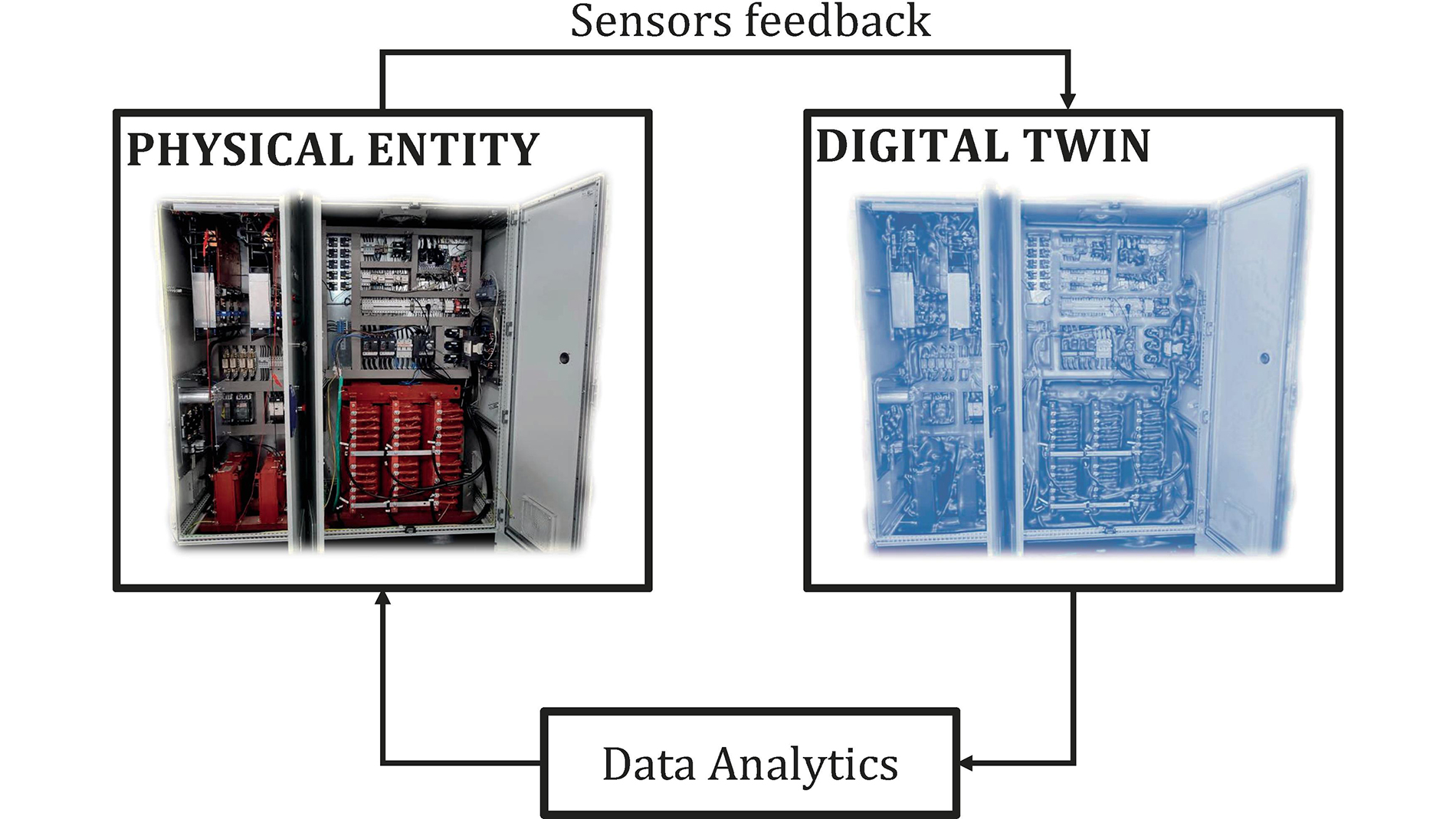
The DT concept (Figure 1), as applied here, allows the creation of virtual models that represent physical systems with high accuracy and real-time response, capturing data from ongoing operations to predict behavior and potential instabilities. The study’s DT-based methodology enables not only stability monitoring but also dynamic control and fault mitigation by continuously updating with real-time data through edge-computing platforms. The paper describes how broadband excitation methods based on pseudorandom binary sequences (PRBS) and Fourier techniques are applied to generate impedance measurements and real-time stability metrics.
Advantages of Digital Twins in power system stability
The integration of DTs enables a real-time view of stability metrics, which is crucial in power systems with parallel inverters due to their continuous dynamic interactions. Traditional stability analysis methods, often based on parametric or nonparametric models, come with significant limitations: parametric models require pre-set parameters that can miss real-time fluctuations, while nonparametric approaches require complex disturbance injections that are impractical for real-time applications. DTs, however, enable adaptive, real-time responses, as they incorporate all physical and control components within a digital replica of the system (Figure 2).
DTs provide a flexible solution for stability analysis by continuously adapting to changing conditions without the need for offline linearization. In scenarios where environmental and system factors are constantly fluctuating, such as in renewable energy sources, DTs offer a method to track real-time stability without disrupting the physical system.
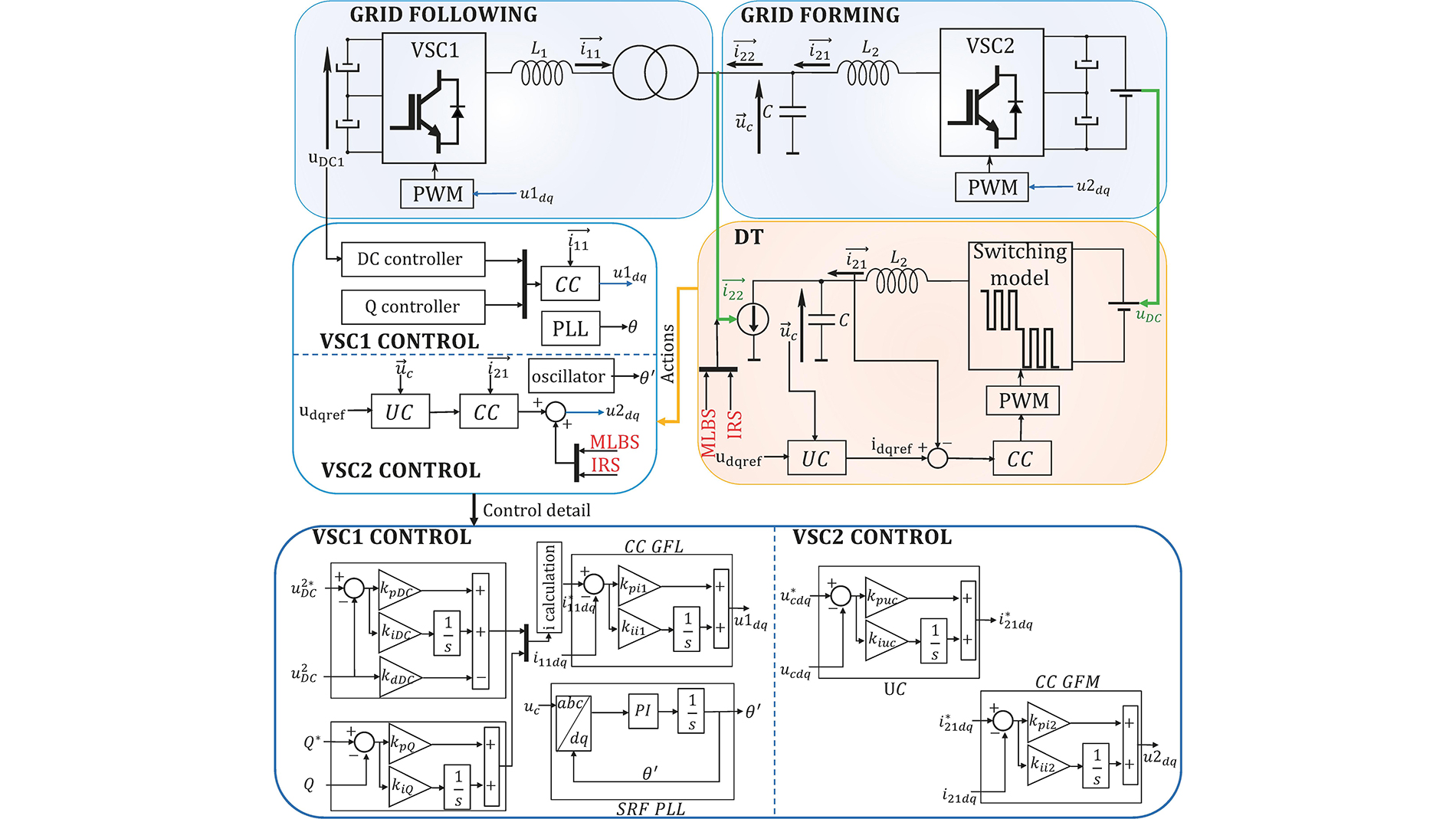
Figure 2: Experimental system setup for the real-time stability analysis based on DT. Image: University of Alcalá/IEEE Xplore
Real-time stability monitoring on edge computing platforms
The DT architecture implemented in this study runs on an edge-computing platform, allowing real-time monitoring of two three-level converters: one grid-forming (GFM) and the other grid-following (GFL) (Figure 3). This architecture is based on a Zynq edge-computing board with dual Arm cores and programmable logic, supporting both the physical system and the digital replica on a single control board. With the GFM converter regulating output voltage and the GFL converter adjusting current to match the grid, the setup effectively illustrates how DTs can monitor and manage complex stability challenges.
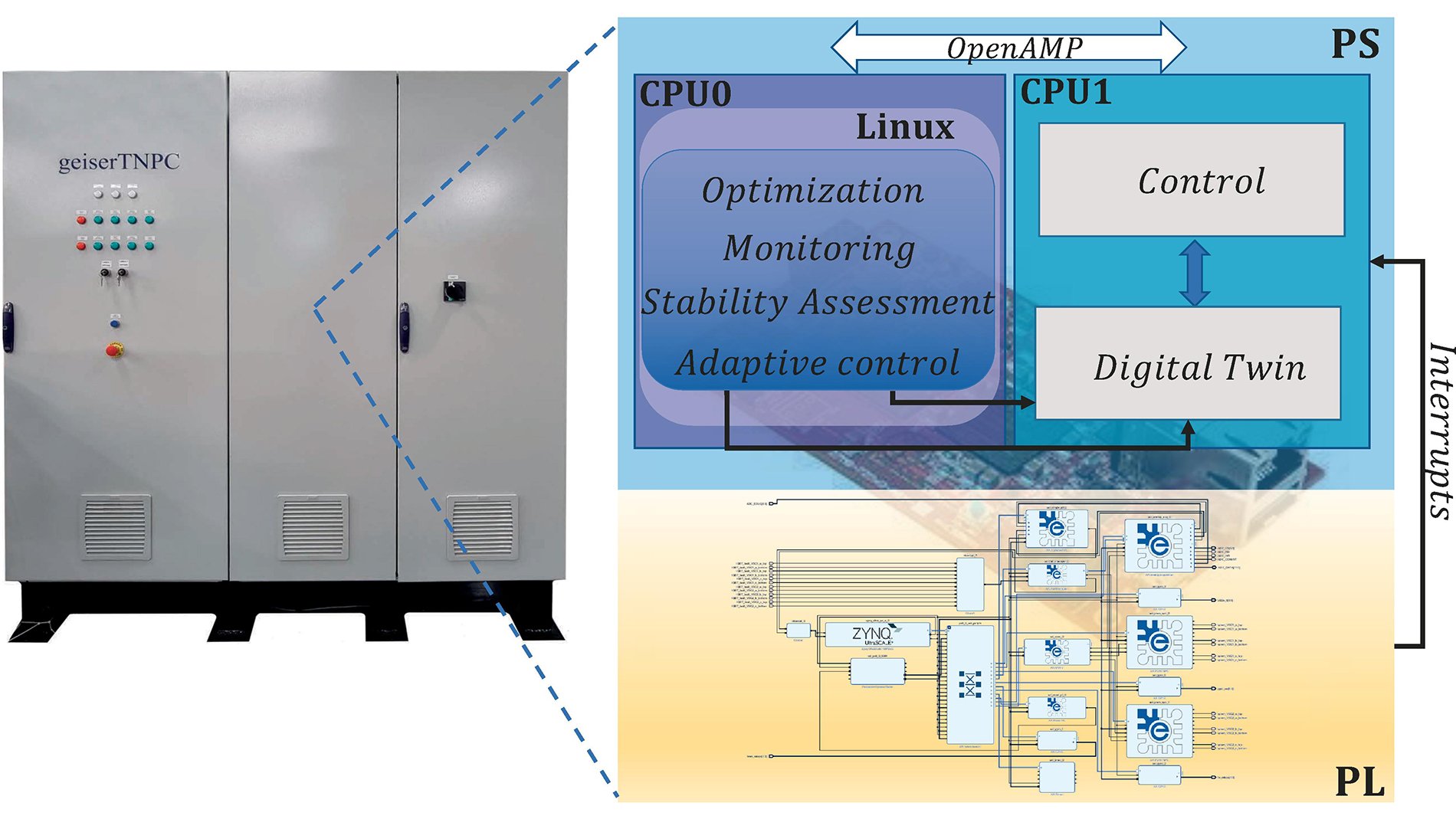
In this setup, sensor inputs from the physical system – such as DC bus voltage and LC filter output currents-feed directly into the DT. Simultaneously, two perturbation sequences are introduced into the DT’s dq channels: Maximum Length Binary Sequence (MLBS) and Inverse Repeat Sequence (IRS). These PRBS signals enhance efficiency by using orthogonal designs that minimize interference and expedite the measurement process. By implementing the DT on an edge-computing platform, the system maintains closely synchronized with real-world operations, enabling accurate and responsive adjustments to control parameters in real time.
Figure 3: Implementation of the DT on an edge-computing platform. Image: University of Alcalá/IEEE Xplore
Digital Twin as a tool for enhanced system stability
This paper demonstrates that a DT can be used to derive real-time stability metrics, such as phase margin, across all feasible operating points of the physical system without needing linearization. The DT’s real-time capabilities support adaptive, online identification of stability parameters, meaning that the DT can respond dynamically to changes in operating conditions. This ability to capture nonlinear effects in real time, such as dead time, conduction, and switching losses, makes the DT approach well-suited for real-world applications.
Key to this capability is the DT’s integration on the same control board as the physical system, allowing simultaneous and consistent control of both domains. This setup facilitates observing how system nonlinearities affect stability, with DT continuously updating the stability analysis based on ongoing measurements from the physical system. Additionally, the DT allows for pre-implementation testing of control adjustments, helping to ensure that changes in the control structure do not negatively impact system stability.
Impedance-based stability analysis with DTs
To estimate the source-to-load impedance, which is crucial for real-time stability monitoring, the DT applies a set of orthogonal perturbations in the dq reference frame. Two types of binary perturbation sequences are used to determine the impedance ratio in both the digital and physical domains, allowing stability analysis based on real-time Nyquist plot monitoring. These sequences (MLBS for the D channel and IRS for the Q channel) are injected into the DT model and physical system simultaneously, allowing stability margin calculation in a single step.
This impedance-based approach enables a comprehensive analysis of the system’s stability by examining the Nyquist plot, which reveals how system stability responds to changes in control parameters. Additionally, this technique enables the detection of specific instability points by applying the GNC to eigenvalues in the characteristic equation of the system. For example, by reducing the GFM converter’s control bandwidth, the researchers could induce oscillations and observe real-time stability degradation, demonstrating the DT’s efficacy in stability prediction.
Technical challenges and optimization
The DT implementation on an edge-computing platform requires several technical optimizations. Given the system’s high-frequency processing requirements, the DT’s time step is set at 2.5 microseconds to ensure that it remains responsive to rapid changes in the physical system. This short time step ensures that the DT can operate synchronously with the physical converter, making it possible to perform stability analyses without delays. However, the platform’s non-preemptive nature and multiple interrupt sources required careful management. In this setup, control interrupts were set at 100 microseconds, with the DT interrupt operating at a higher frequency to minimize the risk of step loss.
Another key optimization is in the design of the PRBS perturbations. These sequences provide high power while respecting amplitude constraints, allowing for an optimal balance between signal-to-noise ratio and real-time accuracy. By applying orthogonal binary sequences, the DT’s measurement time is minimized without losing impedance estimation accuracy. The MLBS sequence has a frequency resolution of approximately 1.2 Hz, and the IRS sequence, being twice as long, provides a detailed frequency response profile across the dq channels.
Real-time experimental validation
The experimental setup for validating this DT approach included two hybrid dual-mode converters: a GFM system with adjustable bandwidth control and a GFL system with fixed parameters. Three test cases were evaluated to demonstrate stability metrics and the impact of bandwidth adjustments on system stability. The experiments showed that reducing the GFM’s bandwidth created conditions for instability, allowing researchers to track stability loss in real time through Nyquist plots and eigenvalue frequency response data.
The DT’s measurements indicated that the phase margin decreased as bandwidth narrowed, with critical instability points detected accurately by the DT analysis. Time-domain analysis showed that as bandwidth dropped, transient response oscillations persisted longer, closely matching the Nyquist-based stability predictions.
Additionally, the DT’s ability to support adaptive control is illustrated by its response to oscillations resulting from PLL-induced instabilities, which arise when the GFM operates within a weak grid. By tracking eigenvalue responses, the DT accurately detected and reported low-frequency phase shifts that indicate impending instability.
Using these real-time metrics, the DT could suggest controller adjustments, such as bandwidth expansions, which were validated within the DT model before implementation in the physical system.
Implications for adaptive control in power distribution
A central benefit of the DT approach is its capacity to support adaptive control by providing real-time stability insights that enable dynamic parameter adjustments. Unlike static gain scheduling, which relies on predefined system conditions, this DT-based approach allows continuous stability monitoring and adaptive control in response to load and system impedance variations. This ability to adapt control strategies dynamically makes the DT approach particularly useful in scenarios with unpredictable grid conditions, such as wind farms or remote installations where grid parameters may vary.
Beyond stability monitoring, the DT enables continuous condition monitoring and fault detection, enhancing system reliability and safety. By consistently evaluating real-time stability margins, the DT can identify patterns that signal emerging faults, providing an opportunity for proactive maintenance. This approach aligns with the growing demand for intelligent monitoring systems in distributed power networks, where stable and effcient power delivery is critical.
Previous research
Already 2022 a paper was published by Elsevier which explores using Digital Twin (DT) technology for monitoring and maintaining the stability of three-phase power converters, essential in the energy transition toward renewable sources. Key components like Voltage Source Converters (VSCs), operating with LC filters to suppress harmonics, are vital for grid integration. The DT approach provides a real-time virtual replica of the physical converter, utilizing edge computing to enable condition monitor ing of LC filters and predicting component wear (Figure 4).
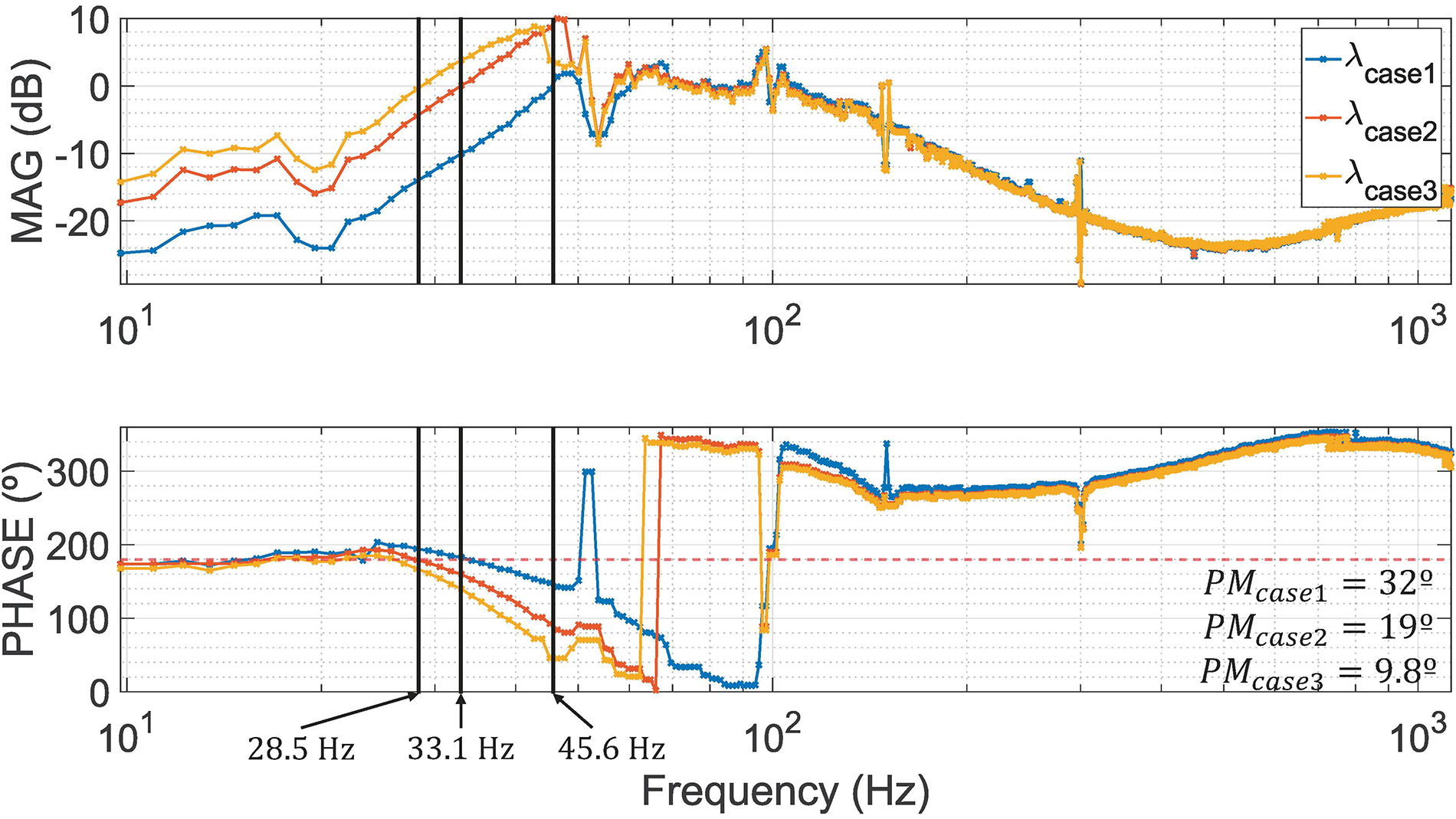
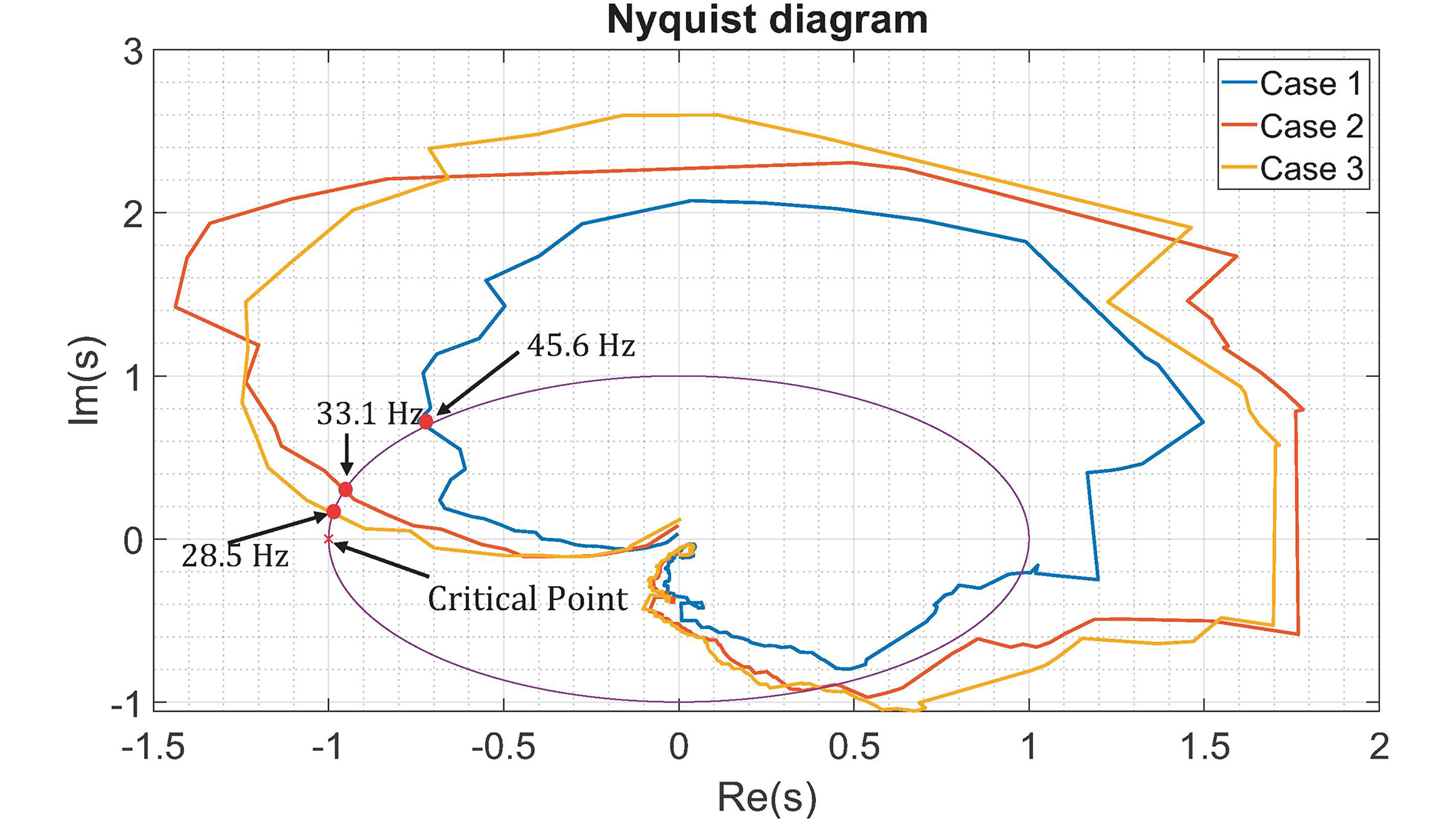
Figure 4: Experimental results of the real-time stability assessment: (a) Eigenvalues of the minor loop of source/load impedance, (b) equivalent Nyquist diagram. Image: University of Alcalá/IEEE Xplore
By incorporating Particle Swarm Optimization (PSO) and Genetic Algorithms (GA), the DT model precisely estimates degradation, supporting adaptive control strategies and predictive maintenance. This setup allows close matching of DT output with real-world signals, enabling proactive adjustments before failures. The study demonstrates the feasibility of deploying DTs in low-cost, edge-computing environments, offering a new avenue for reliable, cost-effcient monitoring and extending the lifespan of power converters. The DT’s successful real-time monitoring on a low-cost platform marks significant potential for broader application across more complex converter systems.
Conclusion
This last study showcases the DT approach as a practical tool for real-time stability analysis and adaptive control in distributed power systems. The experimental results highlight how DTs can offer similar output waveforms to their physical counterparts, demonstrating accuracy in real-time stability analysis. The DT’s ability to work on cost-effective edge-computing platforms underscores its potential for widespread adoption in renewable energy and other distributed power environments.
Future research could expand DT applications to include dynamic stability assessments, such as voltage dips or overvoltages, by training DTs to respond to diverse perturbations. The model could simulate various grid conditions and disturbances, providing comprehensive insights into the converter’s dynamic responses and stability. Additionally, DT technology could further develop to incorporate advanced fault prediction capabilities, creating a fully autonomous stability monitoring and control system.
In summary, this research establishes DTs as a powerful solution for addressing stability challenges in advanced power distribution networks. By providing real-time, high-fidelity monitoring and control, DTs mark a transformative shift in how stability is managed in energy systems, paving the way for the next generation of digitalized, adaptive power networks.